Emanuele Naboni: ‘Digitalisation enables the capture and structuring of complexity, turning every building into a detailed information environment connected to regenerative targets’
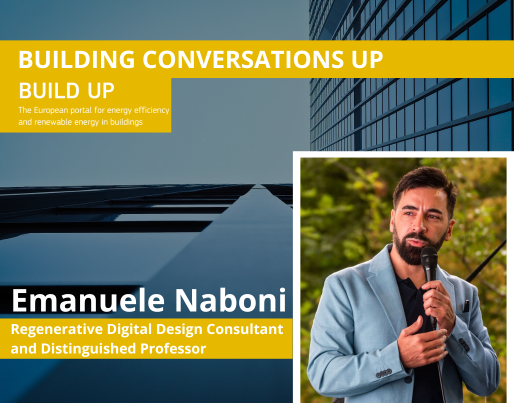
Emanuele Naboni: ‘Digitalisation enables the capture and structuring of complexity, turning every building into a detailed information environment connected to regenerative targets’
Building conversations up with... Emanuele Naboni, Distinguished Professor.
Background
Emanuele Naboni professionally worked with Skidmore, Owings & Merrill (SOM), BIG – Bjarke Ingels Group, Renzo Piano Building Workshop, Kengo Kuma & Associates, William McDonough + Partners, Mario Cucinella Architects, Loisos + Ubbelohde, CITA, Autodesk, Google Technology, Henning Larsen, Velux, Atkins Global, Trenitalia/Italferr, Arup, NASA, the American Department of Energy, and the World Health Organisation (WHO). His contributions span over 130 iconic buildings, 20 master plans across the United States, Europe, Asia, and the Middle East, and 150 full-scale experimental prototypes deployed globally.
In his academic career, he has held professorships at over 15 globally recognised institutions and has lectured at more than 150 universities and research centres worldwide. He is a Distinguished Professor at the University of Seville (Spain). He has served as a Professor at the Royal Danish Academy, the Norman Foster Institute (in collaboration with the MIT Media Lab), the SOS School of Sustainability in Milan, IAAC in Barcelona, EPFL, and ETH Singapore. Initially, he was a postdoctoral researcher at the Lawrence Berkeley National Laboratory.
LinkedIn profile | Instagram profile
BUILD UP (BUP): As a leading figure in parametric and performance-based design, how do you see digitalisation reshaping the way buildings are conceived, designed, and delivered in Europe? In what ways is competitiveness being redefined across sectors?
Emanuele Naboni (EN): Digitalisation transforms European architecture by turning projects into a living, regenerative system, where design goes beyond form-making and is an act of planetary and local repair. Competitiveness belongs to those who digitally embed regenerative restoration, carbon absorption, and human health directly into the DNA of their projects.
The digitalisation of the agenda is evident: 70% of architecture studios utilise 3D rendering platforms, while 40% employ artificial intelligence (AI)-assisted parametric workflows. The concurrent expansion of digital-twin markets, anticipated to reach approximately €300 billion by 2033, provides a data infrastructure for continuous performance verification. Firms that leverage these infrastructural trends create regenerative value and secure financing in alignment with the EU taxonomy and green-bond criteria. These digital (and conceptual) tools integrate geometric design with empirically validated data, yielding systemic solutions that enhance ecological, climatic, carbon, and health conditions, ranging from material assemblies to entire urban ecosystems.
Attaining a competitive edge necessitates proficiency in tools that facilitate systemic regenerative design, enabling designers to embed regenerative logic directly into projects and transform structures, spaces, and infrastructures into contributors to the restoration of planetary and local ecology and health. This approach necessitates agility and interdisciplinarity, integrating experts proficient in computational scripting, environmental data interpretation, and regenerative systems thinking. For instance, in Rome’s Fiumicino train station project, we scripted substantial air volumes, thermal mass for diurnal cycles, natural ventilation pathways through airflow dynamics simulation, and bio-integrated evaporative cooling systems. In Göteborg, we developed climate-adaptive algorithmic frameworks, transforming ecological systems into natural cooling mechanisms for communities and altering building design logic to facilitate this transformation.
This paradigm shift redefines competitiveness. Leadership is demonstrated by delivering built environments that actively contribute to ecological restoration and human well-being, alongside structures engineered for CO2 absorption and optimised performance through digital tools. Competitiveness depends on mastering a new value language: the built form as a systemic organism that contributes net positive value. This involves showcasing ecological literacy, which translates into quantified biodiversity gains; deep carbon intelligence that manages emissions across lifecycles and optimises biogenic storage; and a commitment to salutogenic design, which fosters health through measurable indoor environmental quality metrics.
The New Murabba Plan: A parametric, regenerative framework for Riyadh. In this scheme, the ecological network serves as a digital backbone for controlling microclimates, renewing soil, and promoting local well-being. (Credits: Atkins, Emanuele Naboni)
BUP: Parametric design enables the integration of climate, material, and energy performance criteria from the earliest stages of a project. How does this digital approach contribute to achieving both higher-quality outcomes and greater productivity during the realisation process?
EN: Parametric design enables, from the earliest stages, the encoding of ecological, carbon, and health-related targets into the shape, structure, and assembly logic without deviation; it increases realisation productivity by pre-coding fabrication.
Parametric design functions as an algorithmic framework, encoding complex relationships among climatic and ecological forces, material properties, and necessary ecological and performance metrics into design data. We input essential data into models, including climate change analysis for mitigation and adaptation, ecosystem insights for biodiversity efficiency, material carbon narratives (sourced from lifecycle databases) for decarbonisation and biogenic potential, and health metrics for salutogenic design.
Integrating these scientific and human considerations into an algorithm yields urban and architectural quality that reflects climatic adaptation, ecological reciprocity (as measured by biodiversity metrics), carbon balance (including sequestration potential), and health coherence, all in line with outdoor and indoor environmental quality standards.
‘Parametric design enables, from the earliest stages, the encoding of ecological, carbon, and health-related targets into the shape, structure, and assembly logic without deviation’
Parametric models serve as precise, machine-readable instructions, pre-coding exact tolerances, optimising complex assembly sequences, and generating protocols for advanced manufacturing, such as digital fabrication robotics. I have observed firsthand how this pre-coding transforms realisation processes, significantly reducing errors, accelerating assembly, and minimising waste by optimising material usage — all crucial for achieving regenerative resource efficiency.
Pavilions and 1:1 prototypes in our research serve primarily as exploratory platforms where we test regenerative principles through digital and ecological integration. In Japan, we developed a lightweight timber structure using parametric workflows; the structure embedded site-specific carbon storage modelling directly into its assembly logic. We designed the connections for reversibility, which allowed for material reuse and minimised the long-term ecological footprint. Fabrication relied on granular environmental data streams, aiming to align the physical realisation with local carbon cycles and minimal resource extraction. In further experiments conducted across Italy and other European contexts, we scripted multi-material assemblies using Grasshopper definitions, enabling the direct generation of fabrication protocols for a range of ecological materials, including laminated timber, bio-composites, and fungal-based substrates. We prioritised minimisation of material waste and the embedding of regenerative potentials; biogenic carbon storage and local ecological reintegration guided the design decisions.
Parametric control enabled the environmental performance of these materials to be choreographed digitally. The Milan Student Housing project, developed with Mario Cucinella Architects, extended this regenerative scripting to the urban scale. Environmental datasets informed the parametric development of the massings and façades. Metrics such as thermal variability and induced variability were conceived to promote biodiversity support and cognitive vitality. Digital scripting was used as a continuous framework to engage directly with ecological and climatic complexity; material assemblies, fabrication sequences, and spatial articulations were treated as components of broader regenerative strategies. This digital approach underscores a fundamental connection: the attainment of regenerative quality and enhanced realisation productivity is interdependent when oriented towards regenerative outcomes.

Parametric massing studies for a new student housing complex in Milan investigate how the building can enhance urban biodiversity while adapting to 2030 climate projections.
(Credit: Emanuele Naboni, SOS Mario Cucinella)
BUP: One of the key promises of parametric design is the integration of multiple performance criteria into a single generative model. What role do you envision for AI and digitalisation more broadly in further automating and optimising such multi-objective design processes?
EN: AI enhances regenerative design by collaborating with parametric design systems, creating a synergy in which environmental, material, and health data inform design choices from the outset. Tools such as Grasshopper enable designers to incorporate real-time data regarding climate, carbon, ecology, and human well-being into their geometries; however, manually controlling thousands of variables across varied scales can overwhelm even the most adept teams. AI expands this capacity, enabling the management of complexity, the anticipation of results, and the generation of superior environmental solutions more efficiently and reliably. Designers establish regenerative ecological targets and ethical standards, such as a minimum amount of carbon sequestration, a desired level of cooling, or a specific species richness index. At the same time, AI identifies design options that comply with these criteria within the geometric constraints of the project.
A straightforward example illustrates this well. We developed a prototype for a lightweight timber pavilion designed to reduce local temperatures, capture atmospheric carbon within biogenic materials, and provide shelter for small flora and fauna. AI initially learns from extensive datasets, such as regional climatic patterns, carbon lifecycle data for timber, and ecological site maps. After training, the AI predicted how modifications — such as expanding a canopy or adjusting beam spacing — impact cooling, carbon storage, and habitat development. Within the parametric model, any adjustment made by the designer immediately reveals updated environmental outcomes, fostering a continuous and informed dialogue between human creativity and ecological feedback, where the concept undergoes instantaneous technical assessment.
AI facilitated the quest for optimal solutions. It utilises optimisation engines to systematically explore numerous design variations, ranking them according to performance. Instead of making arbitrary selections, the designer navigates a structured array of alternatives, choosing forms that most effectively contribute to climate adaptation and ecosystem restoration. Machine learning algorithms cluster similar high-performing designs, revealing fundamental principles such as the relationship between surface porosity and microclimate cooling, as well as shading density and habitat quality.
In addition to evaluating and organising information, artificial intelligence can generate entirely new solutions. Utilising generative models trained on regenerative goals, AI suggests structures that may be difficult to envision manually. Advanced interpretive techniques enable designers to comprehensively understand which design parameters have the most significant influence on environmental outcomes. This transparency strengthens the human-AI collaboration.
‘In addition to evaluating and organising information, artificial intelligence can generate entirely new solutions’
The process continues post-construction. Sensors affixed to the pavilion collect empirical data regarding its carbon sequestration capabilities, the effectiveness of its cooling strategies on the surrounding air, and its influence on biodiversity. This data is reintegrated into the model; AI assimilates real-world feedback and refines subsequent projections. Designs evolve as they adapt to environmental realities, rather than relying exclusively on simulations. Buildings transform into dynamic environmental systems that adjust and grow in tandem with the climate, ecosystems, and human communities they support.
This collaborative paradigm revolutionises architectural creativity. Designers establish the objectives, ethical considerations, and regenerative aspirations; artificial intelligence augments their capacity to explore, forecast, create, and modify. Human intent, machine learning, material dynamics, and ecological processes coalesce into a unified design system.
BUP: Digital tools are often underutilised in renovation projects compared to new builds. How can parametric approaches and AI-driven simulations support the deep renovation wave and help mainstream sustainability across Europe’s existing building stock?
EN: Across Europe, the existing building stock accounts for approximately 36% of greenhouse gas emissions and 40% of total energy consumption; yet, less than 1% of buildings undergo deep energy renovation each year. Digital tools remain heavily concentrated in new construction workflows; in renovation projects, 15 to 20% systematically use parametric or performance-driven methods, and the adoption of AI is estimated at less than 5%, according to industry surveys. However, projections indicate that integrating AI-assisted optimisation into renovation workflows could reduce analysis and decision-making time by up to 60%, while improving environmental performance outcomes by 20–30% compared to traditional manual methods based on heuristic evaluations.
Transforming Europe's existing building stock remains essential for achieving climate adaptation, ecological regeneration, and carbon reduction targets. Parametric and AI-enhanced methods provide the structure to unlock this potential, shifting renovation from isolated corrective actions towards a systemic, measurable, and adaptive ecological strategy. Digitalisation enables the capture and structuring of complexity, turning every building into a detailed information environment connected to regenerative targets.
Parametric approaches capture the inherent complexity of existing buildings; 3D scanning, photogrammetry, and archival blueprint extraction enable the creation of procedural digital models where historical geometries, material conditions, and structural logics are encoded as adjustable parameters. Walls, windows, roofs, and load-bearing systems cease to be fixed constraints; they become reprogrammable elements within a flexible computational framework. Each component carries linked environmental attributes: embodied carbon scores, thermal behaviours, biodiversity hosting potential, and health performance indices.
AI enhances this parametric foundation across three primary roles. First, predictive machine learning models trained on renovation datasets anticipate the regenerative performance of intervention options. AI can predict the lifecycle carbon balance of bio-based retrofit materials, estimate the cooling effect of added green surfaces on microclimates, and forecast health improvements associated with enhanced natural ventilation strategies. Second, generative models synthesise retrofit solutions directly optimised for local climates, cultural contexts, and regenerative goals; they generate design options such as bio-integrated façades, hybrid timber-reuse systems, or modular retrofits that maximise resource efficiency. Third, reinforcement learning agents simulate adaptive renovation pathways under future climate projections, exploring dynamic envelope behaviours that reduce overheating risks, support evapotranspiration, and maintain high air quality over time.
Through these mechanisms, renovation becomes a structured field of measurable regenerative opportunities. Integrated AI–parametric workflows allow renovation teams to simulate interventions at multiple scales — façade sections, building envelopes, urban clusters — evaluating operational energy as well as embodied carbon, biodiversity regeneration, evaporative cooling, and human health performance.
Continuous feedback loops close the system, monitoring data from renovated buildings — such as thermal behaviour, carbon retention, air quality, and biodiversity activity — which feed back into predictive models, improving their accuracy and strengthening future project performance. Parametric models adapt dynamically, allowing building stocks to evolve as part of wider ecological and climatic systems rather than remaining static technical artefacts. The systemic adoption of AI–parametric workflows accelerates Europe's renovation wave, translating environmental ambitions into verifiable and scalable actions deeply grounded in regenerative intelligence.
Generative design for renovation shows promising early results, particularly in modular retrofit systems and low-carbon material assemblies, while reinforcement learning applications for adaptive envelopes remain largely experimental. The main barriers are structural: fragmented renovation markets, low levels of digital skills among existing practitioners, limited interoperability between renovation-specific data and AI systems, and high up-front investment costs for complex digital workflows.
‘The systemic adoption of AI-parametric workflows accelerates Europe's renovation wave, translating environmental ambitions into verifiable, scalable actions deeply grounded in regenerative intelligence’
Realistically, achieving full AI-parametric integration for deep regenerative renovation across Europe would require significant scaling of applied research, professional training, and policy incentives to reward regenerative metrics beyond operational energy, as well as the development of user-friendly digital platforms that democratise access to complex predictive and generative tools. Current trajectories suggest that with strategic investments and coordinated effort, a robust partial adoption could be achieved within the next 5 to 8 years, with full system maturity potentially realised by 2040.
BUP: You have worked internationally on pioneering digital projects. Could you share a recent example where the use of parametric tools or advanced digital workflows resulted in a measurable competitive advantage — whether in cost performance, stakeholder collaboration, or other key outcomes?
EN: At the building scale, our work on more than 10 Asian projects leveraged parametric logic to script regenerative facades. These systems incorporated carbon-storing biogenic composites, dynamic shading geometries optimised for seasonal daylight and cooling loads, and bioactive surfaces capable of hosting microbial life for enhanced air quality. Facades achieved up to 34% reductions in peak cooling demands compared to baseline models; exterior biodiversity indices could increase by 80% after five years of monitored growth integration. Material life cycles were closed through digital tagging and reversible assembly strategies, enabling near-complete reusability.
At finer architectural scales, we developed parametric canopies across many global cities through the Master of Architecture programme Extreme Environments at the Royal Danish Academy, where solar-modulating geometries were embedded with evapotranspiration membranes and biological or recycled skin systems that hosted vascular plant species. The best-performing prototypes produced microclimatic cooling of 7–9°C beneath the structures, while sustaining plant life with zero artificial irrigation in temperate summer conditions. These canopies redefined the boundary between built form and living ecosystem. Even at the material level, parametric thinking informed regenerative strategies. Working with advanced ceramic composites, we developed micro-textured facade tiles capable of increasing atmospheric water condensation by 14% in urban heat island zones; modules incorporated integrated seed pockets for spontaneous colonisation by urban flora, supporting habitat continuity across vertical surfaces.
Across these examples, a clear lesson emerges: regenerative performance is scalable, measurable, and creative when parametric frameworks serve ecological and human health objectives directly. Systemic approaches produce interconnected environments where material, climatic, and biological processes co-evolve with built form. These performances emphasise how parametric workflows transform regenerative aims into precise, traceable metrics that directly translate into market advantage. The next leap lies in extending the same rigour beyond project handover. Continuous sensing of carbon flux, microclimate behaviour, biodiversity gain, and material reuse will convert design intent into verifiable environmental evidence, revealing new optimisation cycles. Although current verification protocols remain fragmented, the expansion of sensor networks and transparent data sharing will help bridge this gap, thereby strengthening investor confidence and community trust.
BUP: As we look to the future of the built environment, what key digital skills and design competencies will be essential for tomorrow’s professionals? How can academia and industry collaborate to ensure the sector is equipped for this shift?
EN: When universities invite me to develop new curricula or organisations seek a critical evaluation of their training programmes, I always return to a fundamental question: How can we seamlessly integrate digital mastery with ecological intelligence for designers? My experiences oscillating between architecture schools and practices have taught me one lesson: technical prowess in parametric modelling is valuable when designers comprehend the regenerative processes these models manipulate. Conversely, an understanding of regeneration is adequate when solid digital workflows underpin it.
The intertwined crises of climate change and ecological and health decline in Europe call for a significant recalibration in design education. Future professionals must possess a distinct, cohesive skill set that eliminates the false divide between digital expertise and regenerative intelligence. This signifies an epistemological change; digital tools should serve as the primary language for encoding, simulating, and verifying environmental and social performance at a detailed level.
Attaining this level of digital literacy necessitates an in-depth understanding of data; professionals must be capable of interpreting and integrating various dynamic datasets, from detailed climate forecasts and real-time urban sensor data to material lifecycle inventories, ecological assessments, and even indicators of human well-being.
‘Digital tools should serve as the primary language for encoding, simulating, and verifying environmental and social performance at a detailed level’
At the Royal Danish Academy’s Master of Architecture programme, Extreme Environments, we have continued structuring a pedagogical loop where students write scripts, build a one-to-one fragment, wire it with sensors, stream the data back into the script, rewrite, rebuild, and repeat. Students begin the year by setting up a cloud-based digital twin; the twin carries weather projections, material passports, and carbon factors, while its physical counterpart is nothing more than a plywood frame on the workshop floor. Week by week, the frame mutates. The studio collects prototypes the way a lab collects samples. What matters pedagogically is that students feel both layers. They watch code become climate hardware, then watch hardware send numbers that bend the code. By graduation, they read a Grasshopper slider as a lever on heat flux or CO₂ uptake, and they read a thermistor or strain gauge as a design critique. At the Norman Foster Institute, the integrated curriculum unites design fellows, theorists, and data analysts, underscoring the need for interdisciplinary, systemic design and a redefined relationship between academia, industry, and multiple stakeholders.
In Europe, universities and practices still often operate separately. A proper education in regenerative design requires students to move beyond isolated formal and academic exercises. From the earliest stages, students should be trained to manage dynamic digital twins, connecting geometry, environmental data, and material circularity in a continuous feedback loop with real sites and projects. Mastery of digital tools may be seen differently — not as a technical goal in itself, as often happens. The aim is to establish a continuous flow where academic experimentation and industry practice mutually sharpen each other, grounded in shared evidence and clear environmental objectives. Graduates trained this way will master parametric tools and understand how to structure data, simulations, and physical outcomes together, treating the digital as an active generator of measurable performance. Simultaneously, academic research pushes the boundaries of regenerative potential in industry through computational experimentation. At the same time, industry will be refreshed by becoming acquainted with digital opportunities. In the European context, where climate adaptation and decarbonisation targets are urgent and unavoidable, this integration of digital intelligence from universities, new types of teaching, and environmental accountability is essential.
Examples of digital scripts used to design CO2 absorbing strategies in education. (Credits: Tiago Vasconcelos, David García, Emanuele Naboni)