
Overview Article - Circularity and Low-Carbon Building Materials in Construction
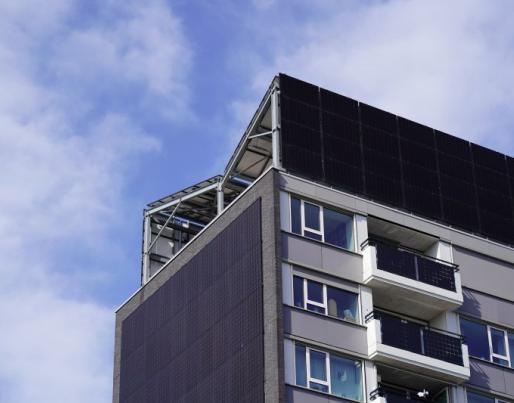
Overview Article - Circularity and Low-Carbon Building Materials in Construction
While the operational energy consumption in low energy buildings is significantly reduced, the embodied GHG emissions of the building materials used in construction can account for half of the life-cycle carbon footprint of the building.
Author: Gianluca Grazieschi (Eurac Research).
Note: opinions in the articles are of the authors only and do not necessarily reflect the opinion of the EU.
The implementation of the principles of the circular economy can significantly reduce the embodied emissions of building materials and is shown by many EU-funded projects aiming at a 50% reduction. This is a significant issue because the construction sector is responsible for more than 40% of the primary energy consumption in Europe, and 36% of the European carbon footprint (Eurostat, 2020).
To tackle this problem, the traditional approach has been based on the application of energy efficiency strategies that are able to significantly reduce the operational energy consumption of buildings. This solution is generally effective, particularly in the case of constructions built in the absence of any energy regulation and with poor construction quality (Asdrubali et al., 2019). Currently, the target of the most ambitious retrofit interventions is that of the Nearly Zero Energy Building, that represents the standard for new construction in Europe. However, when the operational energy demand is reduced and renewable energy systems are integrated in buildings to balance the residual energy requirement, it is possible to detect a significant burden shifting on the embodied energy and embodied GHG emissions (Asdrubali and Grazieschi, 2020).
This is why the attention of this research is focused on low carbon construction materials and the circular economy strategies that can reduce the embodied impacts of building systems and components. In order to support the reduction of the whole-life carbon of buildings the EU has already published several studies and directives, such as the Energy Performance of Buildings Directive (EPBD), the Energy Efficiency Directive (EED), the Waste Management Directive, the Green Product Procurement Directive (GPP), Ecodesign Directive of the Taxonomy Directive (European Commission, 2021, 2020; World Green Building Council, 2022). Furthermore, environmental certification schemes are expanding to support the diffusion of products and design practices that are able to reduce buildings’ embodied emissions: PEF – OEF, EPD, ecolabel, EMAS. In 2018 the European Commission launched Level(s), a common framework to assess the sustainability performance of buildings. Level(s) adopts a life-cycle and circularity approach to guide the construction sector towards environmental sustainability goals. In this regard, the first macro-objective of Level(s) (described as ‘greenhouse gas emissions along a building’s life cycle’) focuses on the operational stage energy performance to reduce the life-cycle Global Warming Potential of buildings.
The second macro-objective of Level(s), (’resource efficient and circular material life cycles’) examines indicators related to the circular utility and efficiency of materials and the measures required to move away from a traditional linear model to shifting the burden on embodied impacts.
This Overview article outlines some approaches European initiatives regarding circularity and low-carbon building materials in the construction sector.
Strategies for low-carbon building materials
The main strategies to reduce the embodied carbon of buildings are listed as follows:
- Design for durability.
- Application of circular economy principles (reduction of waste generation, direct re-use of materials from waste or demolition, use of recycled materials).
- Use of materials with a low carbon footprint (such as bio-based materials) to replace high embodied carbon ones (such as concrete) without concessions on the durability.
- Increase of the environmental performance of the production chains (energy efficiency, renewable energy integration, technological development, industrial symbiosis…).
- Use of local products.
- Design for disassembly and separation at source.
Durability
Durability is a very important aspect because an extended service life of materials or of entire buildings permits the reduction of substitution cycles and prevents waste generation (Grant and Ries, 2013). Design for durability is fundamental in wooden buildings because of the intrinsic weakness of the material when interacting with moisture, rain, and sun irradiation as well as with biological agents, such as termites. Moreover, the corrosion of steel fasteners can represent a significant threat for the reliability of the structure. The decay or deterioration of wooden elements can result in a quick aesthetic deterioration and unserviceability, rather than the collapse of the structure.
Circular Economy Principles
The circular economy principles, mentioned in the new EU Circular Economy Action Plan (2020) which is one of the main building blocks of the European Green Deal (2019), are based on the hierarchy of waste (EU Waste Framework Directive, 2008/98/EC). The hierarchy of waste ranks the management solutions of materials at the end-of-life, aiming at maintaining their economic value in the market if possible and according to what is best for the environment. The prevention of waste is the primary consideration, followed by re-use, recycle, recovery, and disposal (e.g., landfill) is the least preferable solution. The direct re-use of building components is the most interesting solution from the environmental perspective, although its practicability can be restricted in the age of mass production (Stahel and MacArthur, 2019). Some market niches can be detected in limited architectural or design solutions that explicitly adopt a conservative or sensitive refurbishment approach where the re-use of aged wooden beams or bricks can facilitate the conservation of the original appearance of an old building subjected to a renovation intervention. The recovery of construction and demolition waste for the creation of secondary materials is economically feasible and generates energy and climate change benefits (López Ruiz et al., 2020) particularly in the case of some waste flows, such as structural steel, aluminium, and plastics.
On the other hand, the current re-use of mineral waste, which is the main component of construction and demolition debris, is linked to backfilling operations which do not contribute much to the maintenance of the economic value of the materials in the market. Some concerns emerged from the literature about the employment of materials with a high recycled content, and are connected to the slight increase in the costs for the same mechanical performance of concretes (Bostanci et al., 2018), loss of workability (Bostanci et al., 2018), and to the local availability of construction and demolition waste within a close distance (Rosado et al., 2017).
Materials with low-carbon footprint
The use of bio-based materials in building construction is perceived as a good alternative to GHG intensive ones (Grazieschi et al., 2021; Peñaloza et al., 2016) when also considering their carbon capture capabilities. Materials such as wood, soil, clay, cellulose, sheep wool and hemp are expected to play an increasing role in the building sector, although a detailed comparative Life Cycle Assessment (LCA) should be performed to evaluate the consistency of the environmental benefits achievable through their application. One of the most GHG intensive building material is concrete. The production of cement is responsible for about 5% of the global GHG emissions, with around 80-85% of emissions being released during the production of clinker (Huntzinger and Eatmon, 2009). To obtain cement conglomerates with a lower embodied carbon, alternative materials, such as pozzolan, granulated slag, silica fume, and coal fly ash can be mixed in the compound as a substitution to clinker.
Environmental performance of the production chains
Technological development is another factor affecting the impacts attributable to the fabrication of products. The development of the production chains enhances the efficiency of the processes while reducing the input requirements and the generation of waste. Moreover, the exploitation of energy generated through local renewable energy systems can further reduce the non-renewable energy requirement of the process, thereby increasing its climate mitigation benefits.
Local products
The use of local products is a good solution to reduce the impact linked to the transportation from the raw material extraction point to the production site, and from there to the location of construction. This is an interesting strategy, particularly in the case of products that are transported over long distances when the impacts of transportation can be significant.
Design for disassembly
The design for easy disassembly is a useful strategy to separate waste materials at source during the building demolition phase, which avoids additional burdens occurring at the waste treatment plant. The use of dry joints could allow for easier disassembly of building parts, enhancing separation at source, and increasing the recycling potential of materials and components or preserving their residual performance for direct re-use in another construction.
European projects
The EU has funded several projects aiming to reduce of the embodied emissions of building materials, including through the H2020 and LIFE programmes. The following examples focus on the strategies introduced and provide some practical applications.
The ARV project is focusing on the application of prefabricated modular components for building construction and retrofitting as a way to increase the environmental performance of the production stage (see strategy number 4). This strategy can be effective in the reduction of embodied GHG emissions that characterise the construction materials because off-site industrialised production usually generates a lower amount of waste and produces products with a higher performance (due to lower rates of defects of installation, higher precision, and higher quality control, etc.). Moreover, the integration of recycled materials as an input of the production chain (strategy number 2) and the design of elements for easy dismantling at source (strategy number 6) represent further strategies to reduce the embodied burden of building elements while boosting their recovery. Finally, the consideration of local products is supported to limit the emissions from transportation (see strategy number 5). The implementation of these strategies could generate a 50% curtailment in buildings’ embodied energy and related emissions, without increasing the operational energy performance or compromising the indoor comfort.
The BAMB project was concluded in 2019 and targeted the conservation of the value of materials used in buildings when implementing the principles of the waste hierarchy (strategy number 2). Buildings are considered as reversible bodies that can be transformed to host new functions (extending their service life: strategy number 1) or easily disassembled (strategy number 6) to produce material feedstock that can be upcycled in new constructions through ‘material passports’, in which all the information required for their continuous recovery is collected. This proposed approach can save up to 75-90% of waste generation.
EnDurCrete is a H2020 project which ended in 2021 and aimed at reducing the carbon footprint of concrete while enhancing the performance and durability of the traditional composite. The first goal of the project was to apply design for durability principles (strategy number 1) to extend the life span of concrete structures to reduce the production of new cement by studying the factors that cause concrete damage and degradation. Following that, the project started developing innovative low clinker cements and concretes (strategy number 3). The EnDurCrete solution was expected to be 40% more durable than conventional concrete and characterised by a 50% lower global warming potential.
CINDERELA aims to develop a new circular economy model (focusing principally on strategy number 2) connecting industries, construction sector players, municipal services, decision makers and the public in a One-Stop-Shop service. The project targets a 20% reduction of environmental impacts along the value and supply chain. Several other H2020 and LIFE projects focused on the containment of embodied carbon and the application of the circular economy in the built environment include LIFE WASTE2BUILD, CityLoops, CIRCuIT, and HOUSEFUL.
Education and professional training related to the application of circularity strategies and renewable energy systems is very important. In this regard, the BUS-GoCircular project that started in September 2021, is developing and implementing a circular construction skills qualification framework with a focus on multifunctional green roofs, façades and interior elements, while also stimulating market demand for circularity skills.
Circular economy principles are gaining increasing importance in addressing the climate change impacts generated by the construction sector. This is because while the operational energy requirements of buildings are being reduced to low levels and are increasingly balanced by building integrated renewable energy systems, the burden is shifting on to the embodied energy of components.
The strategies to lower the embodied carbon of building components are essentially based on design for durability and disassembly, re-use and recycling, employment of low carbon inputs and materials, technological development of production chains, and the use of local products. The European Union is active on this topic, financing studies and demonstrations to support the application of circular economy principles that can reduce the carbon footprint of the building sector and of the construction materials used within it.
Bibliography
- Asdrubali, F., Ballarini, I., Corrado, V., Evangelisti, L., Grazieschi, G., Guattari, C., 2019. Energy and environmental payback times for an NZEB retrofit. Building and Environment 147, 461–472. https://doi.org/10.1016/j.buildenv.2018.10.047
- Asdrubali, F., Grazieschi, G., 2020. Life cycle assessment of energy efficient buildings. Energy Reports 6, 270–285. https://doi.org/10.1016/j.egyr.2020.11.144
- Bostanci, S.C., Limbachiya, M., Kew, H., 2018. Use of recycled aggregates for low carbon and cost effective concrete construction. Journal of Cleaner Production 189, 176–196. https://doi.org/10.1016/j.jclepro.2018.04.090
- European Commission, 2021. Study on circular economy principles for buildings’ design. Luxembourg.
- European Commission, 2020. Circular Economy - Principles for Building Design. Bruxelles, Belgium.
- Eurostat, 2020. Final energy consumption by sector [WWW Document]. URL https://ec.europa.eu/eurostat/databrowser/view/ten00124/default/table?lang= (accessed 10.27.22).
- Grant, A., Ries, R., 2013. Impact of building service life models on life cycle assessment. Building Research & Information 41, 168–186. https://doi.org/10.1080/09613218.2012.730735
- Grazieschi, G., Asdrubali, F., Thomas, G., 2021. Embodied energy and carbon of building insulating materials: A critical review. Cleaner Environmental Systems 2, 100032. https://doi.org/10.1016/j.cesys.2021.100032
- Huntzinger, D.N., Eatmon, T.D., 2009. A life-cycle assessment of Portland cement manufacturing: comparing the traditional process with alternative technologies. Journal of Cleaner Production 17, 668–675. https://doi.org/10.1016/j.jclepro.2008.04.007
- López Ruiz, L.A., Roca Ramón, X., Gassó Domingo, S., 2020. The circular economy in the construction and demolition waste sector – A review and an integrative model approach. Journal of Cleaner Production 248, 119238. https://doi.org/10.1016/j.jclepro.2019.119238
- Peñaloza, D., Erlandsson, M., Falk, A., 2016. Exploring the climate impact effects of increased use of bio-based materials in buildings. Construction and Building Materials 125, 219–226. https://doi.org/10.1016/j.conbuildmat.2016.08.041
- Rosado, L.P., Vitale, P., Penteado, C.S.G., Arena, U., 2017. Life cycle assessment of natural and mixed recycled aggregate production in Brazil. Journal of Cleaner Production 151, 634–642. https://doi.org/10.1016/j.jclepro.2017.03.068
- Stahel, W.R., MacArthur, E., 2019. The Circular Economy: A User’s Guide, 1st ed. Routledge, New York : Routledge, 2019. https://doi.org/10.4324/9780429259203
- World Green Building Council, 2022. EU Policy Whole Life Carbon Roadmap.