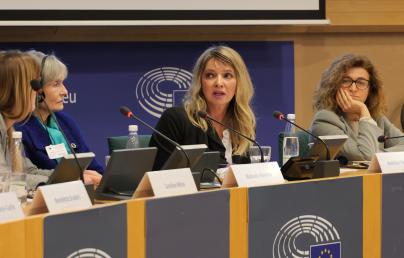
Technical Article - Tool for Building Integrated Photovoltaics (BIPV) early design optimisation
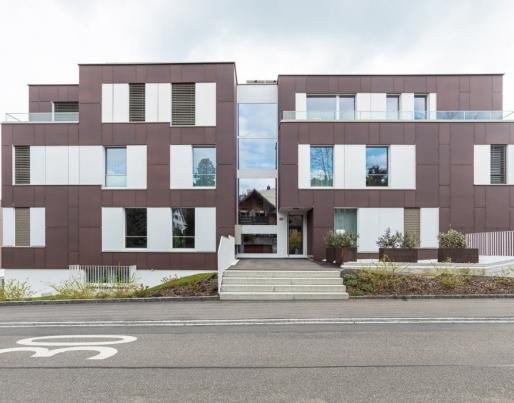
Technical Article - Tool for Building Integrated Photovoltaics (BIPV) early design optimisation
BIPV towards the energy transition
To achieve the objectives set out in the 2030 Climate Target Plan, it is fundamental to accelerate the energy transition through a massive increase of Renewable Energy Sources (RES), energy efficiency and the electrification of many end-use sectors. With the Renewable Energy Directive (EU Directive 2018/2001) on the promotion of the use of energy from renewable sources [1] and the following revision [2], the European Union updated the target for the reduction of Greenhouse Gas (GHG) emissions compared to the levels of 1990, up to 55% compared to the 40% of the previous plan, and the objective to cover at least 40% of the energy demand by RES by 2030. Integrating photovoltaic (PV) technology in the built environment to generate electricity can be one of the main mechanisms towards the global energy transition, and thus the building sector decarbonisation. In 2016 it was estimated that Building Integrated Photovoltaics (BIPV) could technically cover 32% of the building electricity demand at the EU level [3]. A recent study [4] reveals how this 32% is expected to grow by 2030, when buildings in the EU will become more energy-efficient and the efficiency of PV systems will have improved considerably.
Figure 1. Left: example of BIPV façade. Right: detail of the integrated photovoltaic modules. Credits: Sunage SA
Tool for building integrated photovoltaics system optimisation
Introduced as a building component on the roof, façades, or shading elements, etc., BIPV must be properly integrated in the buildings’ structure, overall image and energy system. Considering BIPV at the early stages of the architectural design process, when important decisions on building shape, orientation and location are taken, increases the successful integration from several perspectives, including aesthetic, techno-economic, and environmental. This is the driving force behind a new tool to support architects and other designers to consider the PV integration at the beginning of the buildings’ design, also addressing its expected performance being developed at Eurac Research.
Supported by EU funding through Horizon 2020 EnergyMatching, 4RinEU and Cultural-E projects, Eurac experts developed the BIPV optimisation tool. This tool helps to identify the optimal BIPV configuration, i.e., how many PV modules and where to integrate them over the building envelope (on roofs, façades, shading devices, balustrades, etc.). It can also suggest including an electric storage system to increase the ratio of self-consumed energy. The BIPV configurations are optimised according to the specificities of the cases (considering the buildings’ geometry, local weather, surrounding shadings, unitary costs of the system and current benefits to produce electricity, sold or self-consumed), also considering how much energy is needed by the building throughout the day and the seasons. Different target functions can be assigned to the tool to achieve energy, economic and/or environment-related goals.
Several studies have shown how this approach is distinct from others typically adopted in the common practice of designing PV in buildings and what benefits can be achieved [5], [6], [7], [8].
To summarise, the strengths of the "BIPV optimisation tool" are:
- The optimisation engine that releases designers from performing multiple attempts, simulating and then comparing many different BIPV configurations, with the risk of missing interesting solutions. The optimisation tool starts with subdividing the building surfaces available for PV installation into rectangles representing the PV modules. For the centre of each rectangle the solar irradiation for each hour of the year running a ray-tracing simulation is calculated. The tool also groups the modules by similar orientation and runs the proper optimisation algorithm. During the optimisation process, the algorithm improves the number of modules installed for each group, after calculating the expected hourly production, and at the same time enhances the battery capacity. The optimal solution corresponds to the one that allows the designer to meet the selected target function.
- The fact that the BIPV configuration is the output of the process (not the input).
- The hourly consumption of the building is one of the main inputs and the tool can calculate the hourly matching between PV production and consumption, thus the self-consumed energy.
- The presence of assumptions and the availability of default data, useful in the early design phases, when just basic information about the building is known.
- The possibility to enlarge the evaluation scale to districts, considering energy sharing between buildings.
- The set of Key Performance Indicators (KPIs) provided by the tool for each suggested BIPV configuration, providing insights into the expected performance of the system (related to energy, economic and environment indices), useful support for comparing solutions and taking decisions in a more conscious way. In detail, the available KPIs are: number of battery cycles in the first year, system investment cost (EUR), expected net present value after a specified time period (EUR), expected payback time (years), expected LCOE considering the whole produced electricity (EUR/kWh), expected LCOE considering the self-consumed electricity (EUR/kWh), self-sufficiency (%) (i.e., the portion of energy demand covered by self-consumed electricity only), self-consumption (%), annual cumulative production (kWh), annual cumulative balance production/consumption (taking into account the whole energy produced in the year, also not directly self-consumed), specific emissions of the whole produced electricity (kg CO2-eq/MWh), and specific emission of self-consumed electricity (kg CO2-eq/MWh).
Figure 2. BIPV optimisation tool process, providing a BIPV system configuration and KPIs as outputs. Credits: Eurac Research
Tool application example: combination of a BIPV system with flexible building loads
The BIPV optimisation tool at Eurac represents a useful instrument to support architects and other designers, as well as a way to explore the potential of BIPV with regard to different current topics of research. One of these is the exploitation of the buildings’ flexibility, meaning the capacity of a building to shift its consumption to maximise the use of renewable energy. As presented in the article ‘The role of flexibility in photovoltaic and battery optimal sizing towards a decarbonized residential sector’ [9], the tool was applied to a district-scale residential building case study to evaluate the benefits from the combination of flexible loads and photovoltaic systems with battery storage in terms of energy and environmental indices by optimally sizing the system.
Figure 3. 3D model of the district considered for the application of the BIPV optimization tool. Credits: Eurac Research
Different scenarios with a variable degree of flexibility were created considering various demand profiles, i.e. home appliances, heat pumps, and electric vehicles consumption. The profiles, as well as a list of parameters related to the photovoltaic system and the electricity costs (whose references and calculation methodologies are specified in [9]) have been used as inputs for the BIPV optimisation.
Table 1. Assumptions and inputs considered in the BIPV optimisation
The BIPV optimisation tool was run for each scenario using an economic target function, i.e., to maximise the net present value of the system within the selected time horizon (25 years). The results, in terms of suggested PV nominal power and electric storage (BESS) capacity, self-sufficiency (i.e., the portion of building demand covered by PV electricity directly stored or used in the building) and net present value (normalized with respect to the initial investment) are shown in the following table.
Table 2. Results of the BIPV optimisation
Analysing the results, for Scenario A (lower degree of flexibility) it seems that the most convenient solution in economic terms is to install photovoltaic without battery, as it guarantees an optimal economic outcome of the investment. Nevertheless, the self-sufficiency indicator results are well below the value of the other scenarios, and far away from the targets for the decarbonisation of the residential sector. This is because vehicle charge is done during the night and the heat pumps are not flexible enough to shift part of the night-load during the daytime. The PV system covers only the daytime load of appliances and heat pumps with direct self-consumption.
The results of Scenario B suggest that an infrastructure that allows the charging of electric vehicles during the daytime coupled with batteries can significantly improve self-sufficiency. However, the normalized NPV value is lower compared to the other scenarios, caused by the higher initial investment required to install the optimal nominal power of the photovoltaic system and battery capacity. The contrary effect can be discussed for Scenario C, where heat pumps are considered flexible and 50% of their electric load is shifted during the daytime, there is only a small decrease of the self-sufficiency indicators compared to Scenario B, but such results are obtained with around half the battery capacity. The consequence is a decrease in the initial investment confirmed by the increase in the NPV.
Finally, the results of Scenario D confirm that flexibility coupled with modest storage capacity can lead to good levels of self-sufficiency and be an attractive investment from an economic point of view.
The application of the BIPV optimisation tool allowed for optimal solutions for the given scenarios to be found. It also provided indicators that enabled the comparison and evaluation of different possible combinations of photovoltaic technology and flexible loads. Although the example presented is limited to an energy-economic evaluation (self-sufficiency and net present value), further calculations and analyses were performed and give an insight on the BIPV optimization tool functionalities [9] giving the priority to the role that the residential sector, through the PV - load flexibility combination, can play to achieve the emissions reduction targets set by the 2030 Climate Target Plan.
Thanks to the support of the Horizon 2020 EnergyMatching project, a simplified version of the BIPV optimisation tool is today available on the new online EnergyMatching Platform.
References
[1] European Parliament and Council, “Directive (EU) 2018/2001 on the promotion of the use of energy from renewable sources.” 2018. [Online]. Available: https://eur-lex.europa.eu/legal-content/EN/TXT/PDF/?uri=CELEX:32018L2001&from=EN
[2] European Commission, “‘Fit for 55’: delivering the EU’s 2030 climate target on the way to climate neutrality.” 2021. [Online]. Available: https://eur-lex.europa.eu/legal-content/EN/TXT/PDF/?uri=CELEX:52021DC0550&from=EN
[3] P. Macé, A. El Gammal, D. Mueller, and H. Bürckstümmer, “Technical evaluation of BIPV power generation potential in EU-28,” 2016, pp. 2518–2522. doi: 10.4229/EUPVSEC20162016-6DO.8.6.
[4] H. Gholami, H. Nils Røstvik, and K. Steemers, “The contribution of Building-Integrated Photovoltaics (BIPV) to the concept of Nearly Zero-Energy cities in Europe: potential and challenges ahead,” Energies, vol. 14 (19), no. 6015, 2021, doi: https://doi.org/10.3390/en14196015.
[5] M. Lovati, G. Salvalai, G. Fratus, L. Maturi, R. Albatici, and D. Moser, “New method for the early design of BIPV with electric storage: a case study in northern Italy,” Sustainable Cities and Society, vol. 48, no. 101400, 2019, doi: https://doi.org/10.1016/j.scs.2018.12.028.
[6] M. Lovati, M. Dallapiccola, J. Adami, P. Bonato, X. Zhang, and D. Moser, “Design of a residential photovoltaic system: the impact of the demand profile and the normative framework,” Renewable Energy, vol. 160, pp. 1458–1467, 2020, doi: https://doi.org/10.1016/j.renene.2020.07.153.
[7] J. Adami, M. Lovati, L. Maturi, and D. Moser, “Evaluation of the impact of multiple PV technologies integrated on roofs and facades as an improvement to a BIPV optimization tool,” presented at the 14th Advanced Building Skins Conference & Expo, Bern, 2019.
[8] M. Lovati, J. Adami, M. Dallapiccola, G. Barchi, L. Maturi, and D. Moser, “From solitary pro-sumers to energy community: quantitative assessment of the benefits of sharing electricity,” 2019, pp. 1696–1701. doi: 10.4229/EUPVSEC20192019-6CO.15.4.
[9] M. Dallapiccola, G. Barchi, J. Adami, and D. Moser, “The role of flexibility in photovoltaic and battery optimal sizing towards a decarbonized residential sector,” Energies, vol. 14(8), no. 2326, 2021, doi: https://doi.org/10.3390/en14082326.