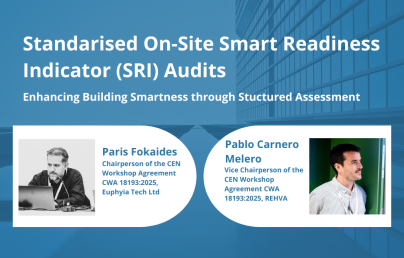
Competitiveness of Integrated Photovoltaics: a case study on residential IPV applications
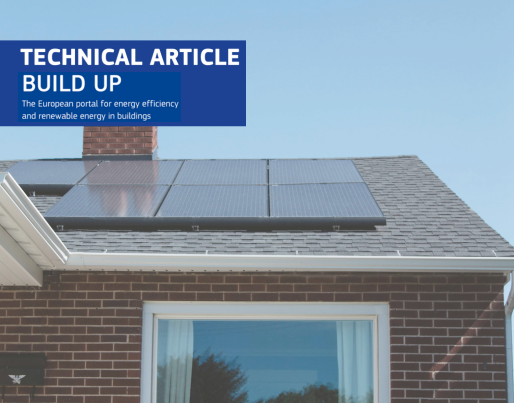
Competitiveness of Integrated Photovoltaics: a case study on residential IPV applications
Authors
Elina Bosch, Senior Analyst, Becquerel Institute, project partner in SEAMLESS PV | LinkedIn.
Philippe Macé, COO, Becquerel Institute, project partner in SEAMLESS PV | LinkedIn.
Ian Kenchington, Analyst, Becquerel Institute, project partner in SEAMLESS PV | LinkedIn.
Delinke Demeter, Marketing & Communication Officer, Becquerel Institute, project partner in SEAMLESS PV | LinkedIn.
(Note: opinions in the articles are of the authors only and do not necessarily reflect the opinion of the EU).
Introduction
The SEAMLESS-PV project is a Horizon Europe Innovation Action that launched in January 2023 and concludes in December 2026. This ambitious initiative, funded by the Horizon Europe research programme under Grant Agreement N°101096126, is composed of research institutes and industrial players covering key roles along the IPV value chain of various sectors: TECNALIA, Mondragon Assembly, Becquerel Institute, PIZ srl, ETA Florence renewable energies, ONYX Solar, CEA, BECSA, BRANKA solutions, Format D2, AKUO Energy, Optimal Computing, Padanaplast SRL, CASA S.P.A., SUPSI, 3S Swiss Solar Solutions AG and CSEM. The project aims to develop advanced PV manufacturing equipment and processes for seamless integration of multifunctional PV solutions, facilitating the deployment of Integrated Photovoltaics (IPV) across various sectors. By focusing on glass-glass lamination and lightweight composite technologies, the project seeks to demonstrate cost-effective, high-efficiency PV solutions tailored to diverse IPV applications, engaging key European partners, and contributing to international IPV standardisation efforts.
SEAMLESS-PV aligns with the objectives of the European Green Deal, which is a comprehensive and ambitious plan proposed by the European Commission to tackle climate change and promote sustainable development across Europe. It was introduced in December 2019 as the EU’s roadmap for transforming the bloc into a climate-neutral economy by 2050. The European Green Deal aims to transform the EU into a modern, resource-efficient, and competitive economy while ensuring no net emissions of greenhouse gases by 2050, economic growth decoupled from resource use, and leaving no person or place behind. The European Commission has adopted a set of proposals such as ‘Fit for 55’ to make the EU's climate, energy, transport, and taxation policies fit for reducing net greenhouse gas emissions by at least 55% by 2030, compared to 1990 levels. All 27 EU Member States committed to this target. [1] [2]
Integrated Photovoltaics: a key technology to reducing greenhouse gas emissions in the building sector
Photovoltaics (PV) emerged as a key technology to reduce net greenhouse gas emissions, and over the past few years the interest for Integrated PV (IPV) solutions has grown significantly. This was driven by a series of factors and trends. First, there is a pressing need to decarbonise various sectors of the economy, including buildings, transportation, and agriculture. Second, there is an equally pressing need to develop renewable energy production in Europe. This has caused the exploration of innovative PV integration solutions. Integrated PV (IPV) systems combine the energy generation function with another function depending on the application, within a single product. This double usage aspect is crucial to IPV, as it aims to reduce overall financial and environmental costs compared to conventional systems achieving the same performance separately. In the context of the construction sector, IPV products combine a building function such as cladding, shading, or watertightness with energy generation. In effect, they act as construction products generating electricity. IPV solutions also offer the advantage of aligning with nearly Zero Energy Building (nZEB) requirements, thereby promoting energy efficiency and environmental sustainability in construction projects. Additionally, the drastic reduction in the cost of PV technology has made it increasingly accessible and financially viable for a wider range of applications, including IPV. Advancements in PV cell and module technologies have been instrumental in enhancing the suitability of PV for integration into various applications, including building facades, roofs, and infrastructure. These developments have led to the creation of more efficient and aesthetically pleasing IPV solutions, further driving the uptake of integrated photovoltaics in both residential and commercial settings.
Building Integrated Photovoltaics (BIPV)
Building Integrated Photovoltaics (BIPV) is steadily gaining adoption and gradually settling as a more mature, recognised and reliable technology. It is mainly driven by in-roof mounting systems using conventional PV modules thanks to specific mounting structures (also referred to as ‘simplified’ BIPV). The BIPV segment has also been pushed by specific and attractive incentives, mostly around a decade ago in France and Italy but also currently in Belgium (Brussels), in Austria and in Switzerland. The integration of PV in buildings can take numerous forms. PV can be integrated into roofs as discontinuous BIPV systems such as in-roof mounting systems, solar tiles, or shingles. They can also be integrated into facades in the form of rainscreen facades or curtain walls. The former are sometimes called ventilated facades, as there is a space between cladding layers for drainage and evaporation allowing for effective water and air barriers, while integrating PV capabilities without significant thermal insulation requirements. The latter are also called non-ventilated or ‘warm’ façades, as their elements are expected to boast thermal insulation capacities. These are often (semi-) transparent, acting as a protective outside layer of the building while ensuring light can enter. Regardless of the integration area, the aesthetic options are wide-ranging in terms of colours, shapes, and sizes thus offering a variety of design options and potential seamless integration in the built environment. The dual function of BIPV (electricity generation unit and construction system) implies that a BIPV installation demonstrates the highest economic and environmental interest at the time of a new construction or a major building envelope renovation. This constrains the BIPV market development to new construction and renovation rates but also allows to leverage the BIPV technology’s double functionality with the potential to meet thermal insulation and renewable energy integration criteria in nZEB requirements. Hence, BIPV emerges as an attractive solution for creating highly sustainable buildings without compromising on aesthetics as demonstrated in many BIPV implantation examples which have become flagship projects.
Barbastro, Spain – Onyx Solar PV ventilated façade
Morbegno, Italy – PIZ multifunctional façade cladding system BIPV
Langenthal – 3S BIPV Roof
Infrastructure-integrated PV (IIPV)
Infrastructure-integrated PV (IIPV) can utilise surfaces that are in the close vicinity of buildings which are often already partially or totally artificial, such as parking lots. In residential areas PV canopies or carports are the main IIPV application. They serve as structures offering shade and protection while generating electricity. They present an enticing opportunity for PV integration, especially in cases where rooftops are not suitable for PV due to aesthetic concerns, or limited available surfaces, such as HVAC systems, windows, chimneys, etc.
Frutigen – 3S Carport integrated PV
Cost benchmark of IPV solutions in residential applications
Based on collected data from SEAMLESS-PV project partners and other external sources (BIPVBOOST project deliverable, BIPV Status report 2020, quotes and publications), the cost of residential IPV solutions was estimated. IPV costs can vary depending on the application, PV system size, module technology, complexity of the mounting system, and aesthetics among other factors. Looking more specifically at the IPV applications considered in this article, BIPV roofing using PV tiles comes at a total system cost between 300 €/m² and 450 €/m².
Rainscreen facades have a slightly higher total cost mainly due to higher installation costs with total system costs ranging from 550 €/m² and 685 €/m² (650 €/m² to 850 €/m² for curtain wall façades). Residential carport integrated PV comes at a total system cost ranging from 1.7 €/kWp to 4.7 €/kWp (1.1 €/kWp to 3.2 €/kWp for commercial carport integrated PV). It should be noted that the cost ranges presented here are the result of a conducted benchmark and do not directly reflect that of the IPV products developed in SEAMLESS-PV project. The cost of these will be evaluated at a later stage in the project. [3] [4]
Extra cost: a notion tailored for IPV systems bearing multiple functionalities
The cost competitiveness is evaluated following a methodology developed during previous research works and tailored to IPV systems bearing multiple functionalities. The methodology is based on the notion of extra costs and correspond to the additional investment needed compared to a conventional construction solution (e.g. investing in a BIPV roof compared to investing in a ceramic tile roof). The extra cost of IPV is mainly the result of electrical hardware, design and installation (inverters, cabling, etc.,), the use of specific fixing and mounting systems, as well as the additional cost of the PV module compared to alternative conventional construction material (ceramic tiles, glass panes, concrete, etc.,). As part of the analysis that was carried out, an average extra cost is assumed per studied IPV application. Extra cost not only varies from one IPV system to another (share of extra cost in total system cost can vary from 40% to 70%) but also for a given IPV solution depending on the project specificities. Consequently, a case-by-case extra cost evaluation is always recommended. [4]
Cost competitiveness assessment results
Based on these extra cost estimations, a competitiveness assessment was conducted. The cost competitiveness is evaluated by weighing extra costs against extra revenues generated by IPV solutions (i.e. generation of PV electricity) using the NPV (Net Present Value) as an indicator. The cost competitiveness assessment is conducted looking at different boundary conditions (irradiation condition, retail electricity price for households, subsidies for (BI)PV), etc.,) which significantly influences the economic viability of IPV solutions. Costs are assumed the same for all countries although some national differences exist, influenced for example by labour costs. The different countries are selected with the aim to represent a variety of conditions.
Figure 1. Cost competitiveness of the BIPV roof solution in three European countries (France, Switzerland and Germany) measured with the NPV in €/m²
In the case of the BIPV roof solution, the cost competitiveness is evaluated in three European countries: France, Switzerland, and Germany. The BIPV system is assumed to have a total installed capacity of 7 kWp, a 30° tilt, a South orientation and a 30% self-consumption rate. Different revenue streams are available in the three countries in addition to savings from self-consumed electricity, such as feed-in tariff, investment support or VAT reduction/exemption. Eventually, the results show positive NPV values. This indicates that the extra investment needed for the BIPV solution (compared to the conventional construction solution) is compensated by the extra revenues allowed by the BIPV solution. The NPV values range from 46 to 133 €/m² depending on the considered country which means that over the course of 30 years (considered lifetime in the calculations) the investment is paid back and an extra 46 to 133 €/m² of profit will be generated. Other IPV solutions used for residential applications such as residential carport integrated PV or ventilated façade in multifamily apartment buildings show similar attractive cost competitiveness results.
This assessment shows that IPV solutions used for residential applications can often be a cost-competitive option. Indeed, in the residential segment, the same drivers as for applied PV solutions can be observed. Firstly, households typically face relatively high retail electricity prices compared to larger consumers in the commercial and industrial (C&I) segment. Secondly, with increasing electrification through at-home electric vehicle charging and electricity-based heating systems like heat pumps, the residential segment shows potential for increasing self-consumption rates. Thirdly, subsidies available for small to medium PV systems further enhance their affordability and attractiveness. Then IPV solutions offer additional benefits, for instance, they lead to savings on construction material costs, particularly evident in BIPV installations where traditional roofing materials like ceramic tiles are replaced. Furthermore, IPV systems can be integrated with insulation layers, enhancing energy efficiency with the potential of reducing heating and cooling needs. Moreover, their aesthetic appeal adds value to residential properties, making them an appealing choice for homeowners seeking both sustainability and visual appeal in their homes.
Conclusion
This article offers a comprehensive overview of the residential IPV landscape, highlighting competitiveness status and drivers. Insights derived from this analysis will contribute to understanding the evolving dynamics and prospects of IPV in buildings. In conclusion, IPV solutions often present a cost-competitive option for residential applications. Factors like high retail electricity prices allowing attractive savings on the electricity bill through self-consumption largely contribute to this cost-competitiveness along with other revenue streams (feed-in tariffs, investment premiums, etc.,). Moreover, IPV solutions provide added benefits like savings on construction material costs and environmental footprint and improved aesthetics, rendering them appealing for sustainable and visually pleasing residential buildings.
References
[1] COM/2019/640final (2019). The European Green Deal. European Commission.
[2] COM/2021/550final (2021). 'Fit for 55': delivering the EU's 2030 Climate Target on the way to climate neutrality. European Commission.
[3] Bonomo P., Bosch E. , et al. (2020), Buildings Integrated Photovoltaics: A practical handbook for solar buildings’ stakeholders. Status Report 2020. SUPSI – Swiss BIPV Competence Centre, Becquerel Institute, in Project BIPVBOOST: Bringing Down the Cost of Multifunctional Building-Integrated Photovoltaic (BIPV) Systems (No. 817991) European Union.
[4] Becquerel Institute, et al. (2020), Competitiveness Status of BIPV Solutions in Europe, in Project BIPVBOOST: Bringing Down the Cost of Multifunctional Building-Integrated Photovoltaic (BIPV) Systems (No. 817991) European Union.