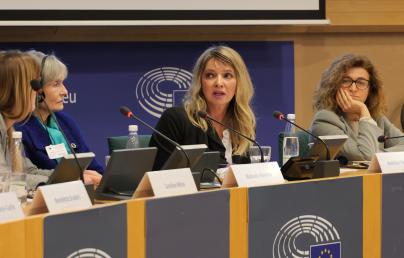
Technical Article – Next generation cooling systems: An analysis of two case studies with active and passive solutions in buildings
Technical Article – Next generation cooling systems: An analysis of two case studies with active and passive solutions in buildings
According to Eurostat data, about 50% of all energy consumed in the EU is used for heating and cooling, and more than 70% still comes from fossil fuels, mostly natural gas. In the residential sector, around 80% of the final energy consumption is used for space and water heating. It is estimated that nearly 20% of the total global electricity demand is used for cooling purposes through air conditioners and electric fans in buildings. Furthermore, the electricity consumption due to cooling is expected to increase due to urbanisation, industrialisation, climate change, and as the standard of living increases globally with some estimates predicting a tripling of the current electricity demand for cooling. [1]
Cooling technologies have been evolving quickly over recently years, due in part to the implementation of several rules at European level, such as the 2016 EU Heating, the Cooling Strategy, or the EU Heat Pump Action Plan (planned for Q4 of 2023). In particular, the 2021 proposal for a review of the Renewable Energy Directive strengthened the heating and cooling target (Article 23) as well as the district heating and cooling target (Article 24).
With regard to the period 2020-2030, the RED II directive could support the development of alternative technologies, such as: vapour compression, vapour compression trans-critical, vapour compression with photovoltaics, solar thermal absorption cooling, waste thermal absorption cooling, membrane heat pump, cold recovery in air handling units/rooftop, cold recovery in building panels, direct ambient air free cooling, indirect ambient air free cooling, use of liquid natural/waste cold source, evaporative cooling, (direct, indirect, or indirect and direct), and cold storage (centralised).
An example of an active system will be described, showing the Cold (Cooling) production facility using a primary system with Ammonia refrigerant (NH3) in an office building located in Madrid with LEED certification with a distribution network of the indirect expansion type in 4 tubes (water-based secondary system). Additionally, an example of a passive system using a cold system based on night ventilation in summer in a theoretical office building in the city of Madrid will be analysed using the DesignBuilder energy simulation software. This system will take advantage of the Mediterranean-continental climate, which presents a high thermal gradient between day and night, making it possible to take advantage of the lower temperatures outdoor than indoor during many summer nights. This condition is particularly useful for encouraging natural ventilation through the selective and motorised opening of windows of the building, equipped with a Building Management System (BMS).
(Note: opinions in the articles are of the authors only and do not necessarily reflect the opinion of the EU)
Authors: Lorenzo Hernando, Ana (Junior Consultant); Corbí Sanchez, Daniel (Project Leader); Fontela Martínez, Miguel (Head of Energy department and Director of Innovation).
Company: NTT Data Green Deal and Sustainable Engineering
Implementation strategies and challenges of renewable energy sources in cooling systems
The most recent application of renewable energy systems as well as thermal energy storage are revised in this article. Optimising the performance of renewable energy systems in buildings is crucial to improving energy efficiency. It is also necessary to integrate renewable energy sources with existing cooling systems. The strategy for the implementation of renewable energy sources, and innovative and more efficient cooling technologies considers three steps:
- Reduce the building thermal energy demand by thermal retrofit (installation of new generation thermal insulation materials for building facades, triple glazed windows, mechanical ventilation).
- Installation of hybrid renewable energy systems, for example: solar-assisted heat pump system with underground thermal energy storage or hybrid PV-wind turbine system coupled with heat pump. Those systems allow a significantly reduced use of primary energy. Both air-source and ground source heat pumps are considered. The air source heat pumps shall be used in a hot climate where the air temperature in winter is no lower than 5º C to achieve a seasonal COP value higher than 3. The ground source heat pumps shall be applied for cold and temperate climate zones, where the air temperature in winter falls below 0º C. In that case, using the underground thermal energy storage or borehole thermal energy storage allows for achieving high values of heat pump COP.
- Space heating (floor heating, wall heating) should be chosen before radiant heating (radiators) to achieve the highest energy efficiency of the heat pump systems.
There has been considerable progress made towards sustainability, however there are still many challenges:
- The RES would be competitive without any or with minimal subsidies at acceptable costs and ROI.
- Minimised full lifecycle GHG footprints, i.e., calculating emissions based only on the operating set point can give a distorted picture.
- Integration of different renewable energy sources (wind, hydro, solar) with thermal and electrical energy storage systems, with grid and back-up energy sources.
- Smart energy management based on efficient integration. Electricity will preferably be synchronised in terms of generation and consumption, and only the surplus of electrical energy generated from renewable energy sources will be stored.
- It is desirable to consume low-cost electricity and electricity with low CO2 (and GHG) emission content, as the emission content of the electricity produced varies over the period of days and weather seasons.
- Developing novel environmentally friendly PCMs such as composite wall systems that could improve thermal comfort and save energy in buildings.
- Efficient integration of PCMs with a solar collector system, photovoltaic system, or thermoelectric installations to combine thermal and electrical energy storage and ensure sustainable energy management.
- Optimisation of buildings with integrated PCMs and PV solar collectors and identification of critical factors affecting the thermal performance of PCM-enhanced buildings with PVT collectors.
- Monitor very rapidly developing and innovative areas closely and implement them where possible.
Practical cases
Active cooling system example
A good example of the use of active systems based on low GWP (Global Warming Potential) refrigerants is the office building No. 1, located in Puerta de las Naciones business complex (4 office buildings in total), developed by Merlin Properties Socimi, S.A. and currently occupied by the pharmaceutical company Roche (Hoffmann-La Roche). Building No. 1 is currently LEED certified.
The air-conditioning system used in this specific building consists of a set of high-performance heat pumps (2 chillers) enclosed into a technical room on the roof of the building, which by means of an indirect expansion system (water) supply cooling to the office spaces via fan coil-type indoor units. The heat is dissipated by cooling towers also located on the roof.
Figure 1. Office Building Nº 1. Source: Merlin Properties
The characteristic feature of this installation is that the refrigerant to be used is ammonia (NH3) instead of more conventional HFC (Hydrofluorocarbon) refrigerants such as R-410 or the more usual R-32. We will analyse this active system with an ammonia-based refrigerant from a variety of sustainability aspects:
Global Warming Potential (GWP)
There are different refrigerants commonly used in the building sector, and from the point of view of environmental impact all of them will generate a Global Warming Potential (GWP) as represented in the following figure:
Figure 2. Comparative Global Warming Potential (GWP) of current refrigerants. Source: NTT DATA
- HFC R-410A: (GWP = 2,088). A mixture of refrigerants, it is still the most widely used in heat pump air-conditioning systems.
- HFC R-32: (GWP = 675). Methylene fluoride, currently the most widely used refrigerant in aerothermal
- R-744: CO2: (GWP = 1). Non-flammable, suitable for direct expansion systems.
- R-290: Propane (GWP = 3). As it is flammable (Class A3 according to ISO 817) it is not suitable for direct expansion systems.
- R-717: Ammonia (GWP = 0). As it is flammable (Class A3 according to ISO 817) it is not suitable for direct expansion systems.
It should be noted that all the refrigerants mentioned above will have an Ozone Depletion Potential (ODP) of 0.
If ammonia is used as a refrigerant, the one with the lowest global warming potential currently available on the market will be chosen. The main problem with ammonia is that it is flammable and it cannot be used in direct expansion systems, i.e., refrigeration circuits that directly cool spaces where people use them regularly.
Impact on sustainability labels
Looking at the BREEAM New Construction Manual 2015, to achieve maximum points in the carbon emission requirements, in the requirement CONT 1. Impact of refrigerants, the impact of CO2 emissions from the refrigerant is rated with a maximum of 2 points. This depends on the degree of CO2 emissions from the refrigerant type.
Table 1. BREEAM NC requirements, CONT 1. Impact of Refrigerants. Source: BREEAM
Table 2. shows the calculation tool CONT 1 (Evidence CONT1.1) which demonstrates that the CO2e EDCV value is 0 kgCO2e/kW, thus achieving the highest level of 2 points in the certification scheme.
Table 2. BREEAM CONT 1 Calculator. Source: BREEAM.
Energy Efficiency Ratio (EER)
The GEA BluAstrum chiller series is especially characterised by minimal maintenance requirements and an extremely slim model design that fits through standard door-sizes. The low dynamic movement of the chiller and the use of touch-proof casings allows for a significant reduction in noise.
Figure 3. GEA BluAstrum 500 chiller technical specifications. Source: GEA Group
Figure 3. shows that the optimal Energy Efficiency Ratio (EER) of 5.0 can be achieved, compared to other refrigeration production systems based on R-410 and R32 refrigerants, which are in an industry average of 4 to 4.5.
Passive cooling system example
An example of a passive system using a cold system based on night ventilation in summer in a residential building near the city of Madrid was analysed using the DesignBuilder energy simulation software. This system takes advantage of the Mediterranean-continental climate, which presents a high thermal gradient between day and night, making it possible to take advantage of the lower temperatures outdoor than indoor during many summer nights. This condition is very useful for allowing natural ventilation through the selective manual and possible motorised opening of windows of the building equipped with a Building Management System (BMS).
For this purpose, an appropriate window design must be carried out so that the maximum amount of glazed area can be opened during the night hours to provide the maximum amount of natural night ventilation.
The case study building is a residential complex consisting of two residential buildings with a height of 5 stories. The building consists of 146 dwellings located in the city of Alcalá de Henares, very close to Madrid (Spain).
The developer is CULMIA Desarrollos Inmobiliarios. To achieve the highest quality standards in its execution, CULMIA faces the challenge relying, among other aspects, on the use of an innovative industrialised construction (structure and facades) with its construction partners AVINTIA (ÁVIT-A system) and NTT DATA in the development of a program of requirements based on the BREEAM EXCELLENT level certification.
Figure 4. Residential building under study. Source: CULMIA Desarrollos Inmobiliarios.
In the city of Alcalá de Henares, summers are short, hot, dry, and mostly clear. The hot season lasts 2.8 months, from 15 June to 10 September, and the average daily maximum temperature is over 29 °C. The warmest month of the year is July, with an average maximum temperature of 33 °C and a minimum of 17 °C.
Figure 5. Annual mean, maximum and minimum temperatures. Source: Weatherspark
To understand the positive impact of night-time free cooling, the building was modelled using the energy simulation software DesignBuilder. To carry out the thermal simulation of the building, the average meteorological data (file ESP_MADRID_IWEC.epw) provided by the DesignBuilder library was used.
Figure 6. Modelling of the building under study by DesignBuilder software. Source: NTT DATA
For this purpose, the geometry of the building was correctly modelled by introducing the thermal transmission values of the building envelope based on better thermal performance values with respect to the existing standards based on a highly insulated and airtight envelope thanks to the industrialised system proposed by CULMIA. The following table characterises the constructive solutions of the building:
Table 3. Construction parameters of the building envelope. Source: NTT DATA
COMPONENT |
DESCRIPTION OF THE SOLUTION ADOPTED |
THERMAL TRANSMISSION COEFFICIENT (W/m2·ºC) |
Roof |
The roofs are non-trafficable with alveolar plate, 14cm thick XPS or rock wool panels, protection, and sun reflective waterproofing sheet. |
0.205 |
Facade |
Double leaf with a prefabricated system consisting of photocatalytic and sun-reflecting paint on a concrete base, EPS insulation (6 cm), concrete panel, rock wool insulation (4.8 cm) and plasterboard lining. |
0.269 |
Exterior Windows |
The exterior carpentry will be of PVC, with thermal bridge break and classification A3/E3/V3. The standard glazing in dwellings will be double glazing with two panes of glass, separated by an air chamber. |
1.200 |
The next step was to analyse the influence of natural ventilation or ‘free cooling’ on the results of the consumption and the cooling demand of the building. This technique not only renews the air inside the building, but also provides free cooling, which reduces the demand for cooling. In summer, buildings tend to accumulate heat inside, but at night, when the outside temperature is lower, this beneficial effect of free cooling can be produced.
The results obtained in the building cooling section are shown below, as well as the total CO2 emissions for the building under study without night ventilation during the summer months (for example, if the tenants use a refrigeration heat pump system).
Comparing these results with those obtained for the same building without free cooling techniques, the reduction in cooling due to the beneficial effect of ventilation is obtained:
Table 4. Comparison of proposed building and building with free cooling. Source: NTT DATA
|
WITHOUT FREE COOLING |
WITH FREE COOLING |
RESULT |
Cooling consumption (final energy) (MWh) |
40.36 |
27.13 |
-33% |
Cooling demand (Use energy) (MWh) |
161.44 |
108.5 |
-33% |
Total CO2 emissions (Tn) |
105.31 |
99.81 |
-5% |
Analysis of the results shows that the effect of free night cooling during the summer months achieves a 33% reduction in both consumption (final energy) and cooling demand of the building. At the same time, the reduction in total CO2 emissions is 5%.
References
[1] International Energy Agency. The Future of Cooling - opportunities for energy efficient air conditioning. Paris: France; 2018.
For more detailed information about sustainable cooling systems, please see the Appendix.
(Appendix - complementary information to the article)
Next generation cooling technologies
Passive cooling strategies
Passive cooling is used to cool a building element without the use of mechanical devices that consume energy. The urban microclimate is an important factor in passive cooling performance [1] [2]. Examples of passive cooling are:
- Improved thermal insulation reduces heat loss to outside in winter and heat gain in summer, improving thermal comfort.
- Ventilated and/or green facades: The first option eliminates thermal bridging and condensation problems by creating a ventilated chamber between the cladding and insulation. In this way excellent thermal performance is achieved, and dampness is prevented. The second is a system in which the building is sheltered from the elements through the use of vegetative cover.
- Trombe wall: a glass panel separated by a dark masonry wall, usually brick, stone, or concrete. The heat of the sun passes through the glass, is absorbed by the thermal mass of the wall, and is then slowly released into the building.
- Façades and roofs designed with high thermal inertia.
- Green roofs, reflective roofs: a system added to the roof of a building to enable the growth of vegetation. Highly reflective, environmentally friendly, and cost-effective roofing system.
- Windows with low thermal transmittance, and with solar shading
- Natural ventilation, night cooling
- Wind towers are one of the traditional passive cooling techniques for natural ventilation. Wind towers have been part of the building geometry in the Middle East for more than three thousand years. Commercial wind towers are divided into quadrants. This allows fresh air to enter and stale (used) air to escape, regardless of the prevailing wind direction. There are two driving forces for the breezeway. The primary force supplies fresh air, driven by windward air pressure, and exhausts stale air, aided by leeward suction pressure. The secondary force is temperature driven and is known as the 'stack effect'. Air becomes less dense as its temperature increases, causing layers of air to stack up. The airflow through the fan is driven by the difference between the internal and external temperatures (micro to macro climate). When the outdoor temperature is lower than the indoor temperature, the buoyancy of the warmer air causes it to rise and be expelled through the unit.
- Evaporative cooling uses water evaporation as a heat source.
- A Solar chimney is an elongated, ventilated cavity painted black to promote the absorption of solar heat. In most cases it is located above the hottest part of the building. The sun's rays heat the air in the chimney, which increases the buoyancy forces that direct the air to the top and then out of the chimney.
- Buried air ducts
- Thermal energy storage
- Phase change materials (PCMs): materials that can store and release large amounts of thermal energy.
Active cooling technologies
If a building cannot be cooled using passive means, active cooling strategies need to be considered. The main cooling systems on the market today are electrically driven compression chillers, with an average coefficient of performance (COP) in the range of 3.0-5.0.
- Heat pumps: Heat pumps are a mature technology that is much more energy efficient than boilers. They allow greater use of renewable energy sources, ambient energy, and waste heat. In buildings, heat pumps are used for heating, hot water, and in some cases also for cooling. There are three main types of heat pumps connected by ducts: air-to-air, water source, and geothermal. Rather than producing heat, they extract and upgrade ambient energy (heat or cold from outdoor air and surface or sewage waters) or geothermal energy (heat or cold from the ground or groundwaters). The refrigeration cycle is in most cases a vapour compression cycle consuming electrical energy, but there are also sorption cycles that are heat driven. [3]
- Thermally driven systems
- Thermomechanical systems: In the thermo-mechanical systems, equipment like concentrating sun-based collectors or evacuated tube collectors (ETCs) is commonly utilised to generate steam. The steam is then processed in the thermodynamic cycle to develop the cooling effect as in ejector systems directly or to produce mechanical power, which drives the prime mover of the vapour compression cycle (VCC) in the case of Rankine and Stirling engines.
- Thermal sorption systems
- Absorption cooling systems: A simple absorption system consists of an absorber, generator, condenser, evaporator, expansion valves, solar heating module, and water-cooling module. In an ABSC system, the refrigerant is fundamentally acting similarly to the refrigerant in the traditional vapor compression system, i.e., it extracts heat from the evaporator due to evaporation while it rejects heat in the condenser due to condensation. However, a thermal compressor is used, not a mechanical one in the vapour compression system. Typical pairs of absorption system working fluids pairs may include a refrigerant like water and either Lithium chloride solutions (LiCl) or Lithium bromide (LiBr) as absorbent; Ammonia (NH3) as a refrigerant and either water, LiNO3, or Sodium thiocyanate (NaSCN) as an absorbent. [4]
- Membrane heat pumps: The current research of the absorption heat pumps is toward to miniaturisation of the components for small scale duty, such as single-family houses, small buildings, or automotive applications [16,17]; however, the conventional heat exchangers used as desorbers, heat and mass exchangers, and desorbers, restrict adopting the absorption heat pump in these fields because are big in size and heavy. In this respect, the membrane devices are a promising technology to surmount the disadvantages of the conventional components. The use of membrane contactors in absorption heat pump systems has been proposed and studied because these devices provide ‘artificial’ interphase between two fluids; therefore, membrane contactors are suitable for the desorption, absorption and heat and mass recovery processes. [5]
- Adsorption cooling systems: A typical adsorption system includes an evaporator, an adsorber, a condenser, a throttle, a heater, and a cooler. The adsorber is packed with solid adsorbent material while the evaporator is charged with the adsorbate material (refrigerant). In the cooling adsorption system, the consequent humidification processes and dehumidification are carried to drive the cycle and develop the cooling effect thermally. However, to keep the cooling effect consistent, the cooling adsorption system either has two beds of adsorbent material to allow concurrent humidification and dehumidification processes in one thermodynamic adsorption cycle and/or is provided with a thermal storage system. [4]
- Dissociative evaporative (or desiccant) cooling systems: DEC is an open system linked to the surrounding air. The desiccant used in DEC systems may be liquid or solid depending on climate condition, operational parameters, and other design constraints. Desiccant cooling is particularly useful for air-based cooling systems since they straightforwardly condition the inlet air into the building envelope. The DECs are most effective in warm and humid areas than the traditional systems, their efficiency improves dramatically in hybrid systems. [4]
Table A1. Summary of innovative active cooling technologies (Adapted from [1])
[3] Single effect
[4] Double effect
[5] Triple effect
[6] Generator absorber heat eXchange
[7] Half-effect
Solar cooling systems: Cooling technologies driven by solar energy have many advantages, including cutting CO2 emissions to decrease global warming, saving heating and cooling bills, reducing the dependency on fossil resources, and reducing imported fuels. Nevertheless, SCACSs are still not penetrating the market due to the high initial and installation costs of SCSCSs compared with the traditional ones driven by electric power (primarily work based on vapor compression cycles), technical considerations in which the traditional systems have a much higher coefficient of performance (COP) than SCACSs, SCACSs are complex (for both the chiller and solar system parts), and experts are required for periodic maintenance and sudden operations, and the limited number of manufacturers and suppliers for SCACSs minimises the competitiveness with the traditional systems. Finally, intermittent availability of solar energy daily and annually requires supplementary storage devices and auxiliary energy systems. Soar energy can be used either by PV modules to provide electricity to the vapour compression module or by solar collectors to deliver the sorption machine at a specific temperature. [7]
Sustainable alternative refrigerants
Refrigerants are another major source of global warming and must be phased out and replaced with lower GWP refrigerants that are non-flammable, nontoxic, and efficient. However, a refrigerant that meets all these criteria does not currently exist. Both synthetic and natural refrigerants are currently being investigated and developed as potential drop-in replacements for existing refrigerants. [17]
Natural cold sources include deep seawater, deep-lake water, aquifer water, lagoon and river water. A water temperature below either 5 ºC (for 100% natural cooling) or 10 ºC (for partial natural cooling) is typically required to achieve a DC supply temperature of 6–7 ºC. [6]
Synthetic/artificial sources from cryogens such as liquefied natural gas (LNG). in support of its GHG emissions reduction goals, the EU Commission now recommends the exploitation of LNG cold energy and waste cold in general to reduce building energy demand. [6]
Ammonia (NH3) The use of ammonia as a refrigerant and absorption cooling is rising, as it is a cheap refrigerant that follows the Montreal Protocol on Substances that Deplete the Ozone Layer and results in efficient cooling systems. Apart from only producing cooling services, an ammonia refrigeration system can be used to provide combined cooling and power. Ammonia is also a valuable energy carrier. It carries energy chemically or through gas liquid phase change. [8]
District cooling (DC)
DC is a collective cold supply system designed for distributing cold, from one or more cold recycling or generation units, via a fluid-based pipe network operated either at temperature levels capable of immediately fulfilling cooling demands of the connected end-users or in some cases, at a temperature level requiring amplification to a lower level at the site of the end-user. The potential of DC systems to contribute significantly to energy conservation, improvements in operational cooling capability, efficiency, flexibility, and reliability, as well as reductions in the environmental impact and cost of building air-conditioning has been well established. [9]
Trends in thermal energy consumption in Europe by 2050 suggest there will be a reduction in the heating demand between 20% and 30%, and a rise of about three times of the cooling demand compared to 2006 values. This tendency is confirmed by the 600% increase of the cooled floor area assessed in the EU between 1990 and 2010. Moreover, the number of space cooling (SC) units installed in the same period, dominated by room air-conditioners, expanded by 24 times with a growth rate of 3% per year. [10]
In 4GDH systems, the same pipes are not able to provide simultaneously both heating and cooling services to different buildings. A 5GDHC network is a thermal energy supply grid that uses water or brine as a carrier medium and hybrid substation with Water Source Heat Pumps (WSHP). It operates at temperatures so close to the ground that it is not suitable for direct heating purposes. The low temperature of the carrier medium gives the opportunity to exploit directly industrial and urban excess heat and the use of renewable heat sources at low thermal exergy content. The possibility to reverse the operation of the customer substations permits to cover simultaneously and with the same pipelines both the heating and cooling demands of different buildings. Through hybrid substations, 5GDHC technology enhances sector coupling of thermal, electrical and gas grids in a decentralised smart energy system.
Figure A1. The four generations of District Cooling (Extracted from [9])
Some research gaps identified for the implementation of the 5th gen DC are increased DC cross-sectorial integrations and synergies to enable exploitation of sustainable and low-cost energy/material flows (including recycled waste/excess flows), more systematic exploitation of locally available renewable electricity/heat supply options and natural/artificial cold sources for direct cooling, and application of more holistic thermodynamic, environmental and economic performance evaluation methods, under an appropriate regulatory framework [10].
References
[1] European Commission, « A Renovation Wave for Europe - greening our buildings, creating jobs, improving lives,» 2020. [En línea]. Available: https://eur-lex.europa.eu/legal-content/EN/TXT/?qid=1603122220757&uri=CELEX:52020DC0662. [Último acceso: Febrero 2023].
[2] R. Ismaen, T. Y. ElMekkawy, S. Pokharel, A. Elomri y M. Al-Salem, «Solar Technology and District Cooling System in a Hot Climate Regions: Optimal Configuration and Technology Selection,» Energies, vol. 15, p. 2657, 2022.
[3] P. Chauhan, S. Kaushik y S. Tyagi, «Current status and technological advancements in adsorption refrigeration systems: A review,» Renewable and Sustainable Energy Reviews, vol. 154, p. 111808, 2022.
[4] C. Díaz-López, A. Serrano-Jiménez, K. Verichev y Á. Barrios-Padura, «Passive cooling strategies to optimise sustainability and environmental ergonomics in Mediterranean schools based on a critical review,» Building and Environment, vol. 221, p. 109297, 2022.
[5] S. Buffa, M. Cozzini, M. D’Antoni, M. Baratieri y R. Fedrizzi, «5th generation district heating and cooling systems: A review of existing cases in Europe.,» Renewable and Sustainable Energy Reviews, vol. 104, pp. 504-522, 2019.
[6] C. S. Meena, A. Kumar, S. Jain, A. U. Rehman, S. Mishra, N. K. Sharma, M. Bajaj, M. Shafiq y E. T. Eldin, «Innovation in Green Building Sector for Sustainable Future,» Energies, vol. 15, p. 6631, 2022.
[7] J. D. Hunt, A. Nascimento, B. Zakeri, P. S. Franco-Barbosa y L. Costalonga, «Seawater air-conditioning and ammonia district cooling: A solution for warm coastal regions,» Energy, vol. 254, p. 124359, 2022.
[8] Q. Al-Yasiri, M. Szabó y M. Arıcı, «A review on solar-powered cooling and air-conditioning systems for building applications,» Energy Reports, vol. 8, pp. 2888-2907, 2022.
[9] S. Zhang, P. Ocłon, J. J. Klemes, P. Michorczyk, K. Pielichowska y K. Pielichowski, «Renewable energy systems for building heating, cooling and electricity production with thermal energy storage,» Renewable and Sustainable Energy Reviews, vol. 165, p. 112560, 2022.
[10] L. Kranzl, P. Mascherbauer, M. Fallahnejad, S. Pezzutto, A. Novelli, A. Zambito, P. Miraglio, A. Belleri, L. Bottecchia, S. Gantioler, P. Riviere, A. Etienne, P. Stabat, T. Berthou y J. Viegan, «Executive summary of the study “Renewable Cooling under the Revised Renewable Energy Directive ENER/C1/2018-493”,» European Comission, 2021.
[11] S. Garimella, K. Lockyear, D. Pharis, O. E. Chawa, M. T. Hughes y G. Kini, «Realistic pathways to decarbonization of building energy systems,» Perspectives Joule, vol. 6, pp. 956-971, 2022.
[12] IEA (International Energy Agency), «The Future of Heat Pumps: World Energy Outlook Special Report,» 2022.
[13] J. Ibarra-Bahena, S. Raman, Y. R. Galindo-Luna, A. Rodríguez-Martínez y W. Rivera, «Role of Membrane Technology in Absorption Heat Pumps,» Membranes, vol. 10, p. 216, 2020.
[14] P. A. Østergaard, S. Werner, A. Dyrelund, H. Lund, A. Arabkoohsar, P. Sorknæs, O. Gudmundsson, J. E. Thorsen y B. V. Mathiesen, «The four generations of district cooling - A categorization of the development in district cooling,» Energy, vol. 253, p. 124098, 2022.
[15] V. Eveloy y D. S. Ayou, «Review. Sustainable District Cooling Systems: Status, Challenges, and Future Opportunities, with Emphasis on Cooling-Dominated Regions,» Energies, vol. 12, p. 235, 2019.
[16] R. A. Almasri, N. H. Abu-Hamdeh, K. K. Esmaeil y S. Suyambazhahan, «Thermal solar sorption cooling systems - A review of principle, technology, and applications,» Alexandria Engineering Journal, vol. 61, pp. 367-402, 2022.
[17] https://www.energy.gov/eere/buildings/road-zero-does-next-generation-heating-and-cooling-rd-strategy