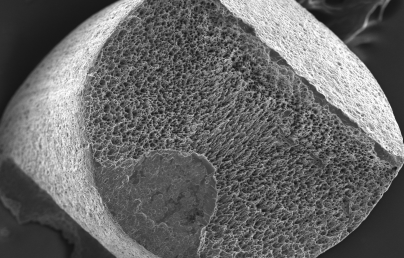
Technical Article - From opaque traditional walls to Adaptative Façade Systems: state of play and outlook
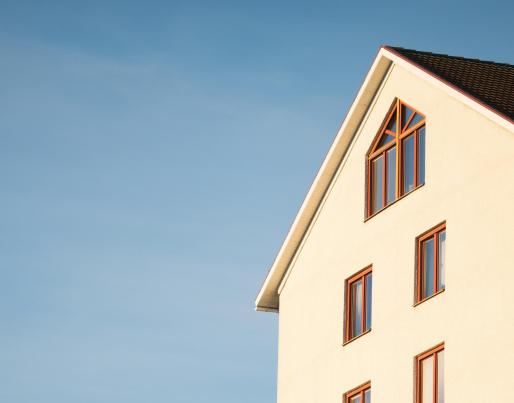
Technical Article - From opaque traditional walls to Adaptative Façade Systems: state of play and outlook
Authors:
Miren Juaristi, Davide Brandolini (Institute for Renewable Energy, Eurac Research).
Note: opinions in the articles are of the authors only and do not necessarily reflect the opinion of the EU.
For a better understanding of this article, EURAC has also prepared this infographic on opaque façade systems. Check it here.
What makes a façade intelligent?
Opaque façade technologies have incrementally evolved both in new construction a by increasing their thermal insulation and airtightness levels aiming to reduce the overall energy demand from the buildings. These envelope systems have now become conventional and are designed to guarantee comfort for average annual conditions. Nevertheless, to face the climate change crisis and to further reduce buildings’ energy consumption, building envelopes must have an optimal performance during each environmental condition. Therefore, adaptive facades are considered one of the most promising technological options, as they can autonomously and reversibly modify some of their functions, characteristics, or behaviours under different boundary conditions[1]. When the adaptive behaviour of the façade is managed by an external control system (through sensors, actuators, and computer algorithms) this is an intelligent façade, which optimises the buildings’ performance in a dynamic and controlled way.
Today, the built examples of intelligent façades have a dynamic behaviour only in the transparent part of the envelope. The autonomous control of solar thermal gains and natural lighting, through smart windows systems and mobile shading elements, is in fact the most applied dynamic behaviour in building envelopes. Smart curtain walls are already a technological reality and there are more complex variants than simple light control. For example, in double-skin curtain walls, both the shading and the ventilation openings of the inner skin are controlled. In this way, the building can be naturally ventilated when the air in the chamber is at the desired temperature. In addition, by controlling the openings of the air chamber, the temperature can be regulated by heating up the intake air on sunny winter days exploiting the greenhouse effect or preventing overheating in summer by ventilating the air chamber to the outside.
Over the last decade, new technological concepts have been emerging to extend the adaptive behaviour throughout the whole façade, such as adaptive opaque façades. By integrating dynamic, adaptive, or intelligent functioning into the opaque façade components, the exchange of thermal energy is allowed or blocked under different weather conditions and building uses. Thus, the behaviour of the façade becomes optimised for each circumstance.
Integrating intelligent natural ventilation with opaque façade technologies, a first approach with limitations for a market uptake
The exchange of air between the indoor and outdoor environment is one of the mechanisms to control the heat flow through the building’s envelope. For example, warm indoor air could be extracted during periods of overheating, reducing the demand for cooling. The demand for heating could also be reduced if the outside air was pre-warmed in the ventilation chamber of the façade, before being injected inside. In all the described cases, it is necessary to generate a pressure change between the external and internal environment, to transport the air. Therefore, these walls are equipped with electric fans connected to a control system that ensures the intelligent operation of the system.
Both the parietodynamic and permeodynamic walls work through this mechanism. In parietodynamic walls the air is transported through a dedicated channel that connects the exterior and interior space. In permeodynamic systems, the air passes through the façade materials, thanks to the difference in pressure between the two environments. These walls have been prototyped and tested in the research environment[2] , but have not successfully reached the market due to some of their limitations[3].
One of the limitations of the parietodynamic wall is its acoustic performance; the duct inevitably represents an acoustic bridge. In such a case, natural ventilation could be a similar bioclimatic strategy in terms of its ability to dissipate internal heat gains and therefore the added value of the complexity of the parietodynamic wall is not clear. The permeodynamic wall does not present this problem, but its durability could be compromised by the permeability that the materials used should have, both to water vapour and to particles present in the air, which would end up clogging the pores of this breathable component.
It must be also specified that, although the intelligent control of natural ventilation may be an adequate strategy to reduce the cooling demand of our buildings, this strategy could be inadvisable in urban environments where high levels of environmental and acoustic pollution are common. Moreover, it might be difficult to integrate the adaptive behaviour in renovations.
Next generation of Adaptive Opaque Façade technologies
What if the opaque façade worked as a removable coat for the building?
Dynamic Insulations or Active Insulation Systems
Imagine a conventional ventilated façade, exterior cladding, an air chamber of about 5 cm, mineral wool insulation, and an internal layer of brick, supported by concrete slabs. Mineral wool offers a thermal resistance that aims to delay the exchange of energy between the interior and exterior environment by default. As anticipated above, several studies[1], [4], [5], [6] have demonstrated that a significant amount of energy could be saved by dynamically varying the thermal conductivity of the insulating layer.
For example, during colder seasons the thermal resistance of the insulating layer during the hours when solar irradiation is directly hitting the building’s envelope could be reduced (i.e., the building coat might be ‘removed’ during those hours). In this way, the heat absorbed by the irradiated building’s facades would then be transmitted through the wall in the indoor environment thanks to the reduced thermal proprieties of the insulating layer. By achieving situations like this one, a significant amount of energy could be saved, reducing the overall required energy needed to maintain the desired indoor temperature.
To obtain this adaptive behaviour, the mineral wool layer should be replaced by a dynamic insulation layer. Several technological concepts have been studied in the decade and are still under development, such as gas-filled panels, closed-loop dynamic insulations, and movable multi-layer panels. All these elements can change their thermal transmittance modifying their internal configuration.
Gas-filled panels present a very low thermal transmission when the panel is emptied of this gas (which passes into an adjacent tank) while increases when the panel is refilled with the gas. Multilayer panels are similar to accordions; they are made up of several layers of aluminium separated by air chambers. When the ‘accordion’ is open, the succession of these air chambers limits the thermal exchange between two environments. By closing the ‘accordion’, the aluminium layers are in contact, and thanks to conduction, they dissipate thermal energy effectively.
Another technological concept uses movable insulation components as a Dynamic Insulation System and was proposed by researchers of the University of Colorado. This concept consists of an insulation layer which is enclosed within two fixed layers. Between the insulation layer and inner and outer enclosures, there are closed air cavities. The insulation layer is divided into several pieces, which can rotate simultaneously if actuated by mechanical actuators. When the insulation layers are aligned and in contact, the system acts as conventional insulation. When the layers are rotated instead, they enable convective movements between the two air cavities and decrease the thermal resistance of the system. The maximum heat transfer in the system is achieved when the pieces of the insulation layers are parallel among them. As far as the authors know, only the concept patented by Krarti has been tested in a laboratory so far to characterise its dynamic thermal behaviour[7].
Figure 1 Dynamic Insulation System prototype at Larson Building System Laboratory at the University of Colorado, Boulder. Concept patented by Krarti and tested in a laboratory to characterise its dynamic thermal behaviour[7].
For the other technology concepts, their effectiveness has been evaluated through dynamic simulations in certain climates and building typologies[4]. Although these results should be validated with corresponding experimental tests, everything indicates that they effectively reduce the thermal consumption of buildings. It is essential to have well-designed control algorithms and a wide range of adaptations, i.e., guaranteeing high thermal insulation when necessary and effectively exchanging heat in suitable boundary conditions.
Kinetic claddings
Another way to convert opaque façade systems into intelligent systems is by replacing traditional fixed cladding with mobile cladding[8]. The objective is to be able to control the thermal gains due to incident solar radiation. The mobile cladding would have two possible geometric configurations, from which two different solar absorptances would derive. The mechanism of this mobile cladding would be similar to one seen with mobile shading elements, with sensors which monitor external and internal conditions, actuators which allow changing from one geometric configuration to another, and a control system that decides which configuration is the right one according to the boundary conditions. The solar absorptance control does not seem to have enough impact if it is applied in conventional façade systems. However, energy consumption is substantially reduced when the mobile cladding is combined with the integration of dynamic insulation and control capable of adequately managing the adaptation of thermal gains by radiation and heat transfer through the enclosure.
Controllable ventilated air cavity
An additional component of the conventional ventilated façade that could become intelligent is the air chamber[8]. The convective movements that occur in the air chamber leads to the dissipation of part of the thermal energy that is being exchanged between the interior and exterior environments. These convective movements depend on various factors: the thickness and geometry of the air chamber, the configuration of the cladding joints, the temperature gradient between the lower part and the upper part of the air chamber, solar radiation incident, and the speed and direction of the wind. The greater the convection in the air chamber, the greater the thermal dissipation that occurs in it.
Adaptive opaque façades might represent the future for lowering buildings’ energy use and carbon footprint
All the previously cited studies seem to indicate that energy optimisation is greater when more than one physical phenomenon can be simultaneously controlled, considering not only current situations but also past conditions and future forecasts. An intelligent building could thus optimise its energy consumption by integrating intelligent glass and adaptive opaque façades, capable of controlling its solar absorptance, convective movements in the air chamber, and the flow of heat through its thermal insulation. It might seem that the sophistication of the opaque façade is an inexorable stumbling block for its massive application since current solutions are cost-effective, simple, and require little maintenance to ensure high durability.
However, in certain applications (suitable climates and building typologies) and combining them with other bioclimatic strategies the integration of these technologies could significantly reduce or even eliminate the demand for heating and cooling. This is the true objective of intelligent façades and if the research manages to find the keys for its optimisation and demonstrates its environmental suitability not only during the use phase of the building but throughout its life cycle, they will cease to be iconic buildings and will become the new constructive paradigm. However, their technology readiness is still too low, mostly because previous research activities were scattered and isolated experiences. To reach needed technology maturity, interdisciplinary research and development for the next generation of Adaptive Opaque Façade technologies will be certainly needed over the incoming years.
With this purpose and interdisciplinary team, a new EU-funded project called ZERAF will kick off in 2023. This project is co-ordinated by the Italian private research centre Eurac Research and will have as partners Indresmat SL, Ingpuls Smart Shadings GmbH, Active Insulation System B.V, and Technische Universiteit Eindhoven. ZERAF will directly develop, test, and work for the future market uptake of an intelligent opaque façade technology for both new and existing buildings.
According to the promising preliminary but non-validated results, the buildings’ operational energy use could be reduced by up to 69% with advanced control strategies (for specific climates, building characteristics and urban contexts) [5]. ZERAF aims at scientifically proving such potential. If succeed, considering the size of the EU building stock where ZERAF could be applied, it is estimated that by developing a technology focused on modularity and adaptability to diverse contexts between 2030 and 2050 the cumulative thermal energy use of the European building stock might be reduced by 1.4 TWh compared to currently available high-performing façade and HVAC systems [9]. This means that in 2050, residential buildings across the EU could save up to 125 GWh electricity per year thanks to ZERAF technology (under the assumption of space heating and cooling provided only by heat pumps).
Substantial economic savings in energy bills of final users are expected. In fact, considering the mean electricity prices of 2018 for the EU households and average thermal consumptions, the end-user of a residential building with ZERAF technology could pay 1 €/m2 per year for the electricity needed for thermal energy. Another positive effect would be the minimisation of operational energy use in buildings, the reduction of the total amount of greenhouse gas emissions, and hence the Global Warming Potential (GWP). If ZERAF technology is proofed to perform according to the most optimistic predictions, a reduction of 1065 million of tCO2eq would be possible compared to what would have been emitted if high-performing façade and HVAC technologies were applied in renovations and new buildings constructed between 2030 and 2050 [10].
ADJOURNED REFERENCES
[1] R. C. G. M. Loonen, M. Trčka, D. Cóstola, and J. L. M. Hensen, “Climate adaptive building shells: State-of-the-art and future challenges,” Renewable and Sustainable Energy Reviews, vol. 25, pp. 483–493, Sep. 2013, doi: 10.1016/j.rser.2013.04.016.
[2] M. Fawaier and B. Bokor, “Dynamic insulation systems of building envelopes: A review,” Energy Build, vol. 270, p. 112268, Sep. 2022, doi: 10.1016/j.enbuild.2022.112268.
[3] M. Juaristi, T. Gómez-Acebo, and A. Monge-Barrio, “Qualitative analysis of promising materials and technologies for the design and evaluation of Climate Adaptive Opaque Façades,” Build Environ, vol. 144, pp. 482–501, Oct. 2018, doi: 10.1016/j.buildenv.2018.08.028.
[4] S. J. M. Koenders, R. C. G. M. Loonen, and J. L. M. Hensen, “Investigating the potential of a closed-loop dynamic insulation system for opaque building elements,” Energy Build, vol. 173, pp. 409–427, Aug. 2018, doi: 10.1016/j.enbuild.2018.05.051.
[5] Q. Jin, F. Favoino, and M. Overend, “Design and control optimisation of adaptive insulation systems for office buildings. Part 2: A parametric study for a temperate climate,” Energy, vol. 127, pp. 634–649, May 2017, doi: 10.1016/j.energy.2017.03.096.
[6] M. Juaristi, F. Favoino, T. Gómez-Acebo, and A. Monge-Barrio, “Adaptive opaque façades and their potential to reduce thermal energy use in residential buildings: A simulation-based evaluation,” J Build Phys, vol. 45, no. 5, pp. 675–720, Mar. 2022, doi: 10.1177/17442591211045418.
[7] M. Dabbagh and M. Krarti, “Evaluation of the performance for a dynamic insulation system suitable for switchable building envelope,” Energy Build, vol. 222, p. 110025, Sep. 2020, doi: 10.1016/j.enbuild.2020.110025.
[8] M. Juaristi, A. Monge-Barrio, A. Sánchez-Ostiz, and T. Gómez-Acebo, “Exploring the potential of Smart and Multifunctional Materials in Adaptive Opaque Facade Systems,” Journal of Facade
[9] Calculated from R. Pernetti, R. Pinotti, and R. Lollini, “Repository of Deep Renovation Packages Based on Industrialized Solutions: Definition and Application,” Sustainability, vol. 13, no. 11, p. 6412, Jun. 2021, doi: 10.3390/su13116412.
[10] Calculated from L. Hildebrand, “Strategic investment of embodied energy during the architectural planning process,” 2014.